Bio: Randolph E. Kirchain, Ph.D.
Director, MIT Concrete Sustainability Hub
Principal Research Scientist, MIT Materials Research Laboratory

Summary
Dr. Kirchain’s research and teaching explores the impact of materials-technology decisions on the economic and environmental performance of the products into which those materials are transformed and the systems in which they are produced, used, and eventually discarded.
The choice of material potentially has sweeping implications on the realization of a product. Materials dictate available production processes, and therefore the physical constraints within which a designer must work. Similarly, the synergism of design, materials, and process affect the environmental impacts associated with a product’s manufacture, its use, and its ultimate disposal.
As such, understanding the implications of a materials-technology decision requires characterizing the effects that occur throughout every stage of the material/product life-cycle.
To address this, Dr. Kirchain’s research deals with the development of methods to model two critical aspects of technological performance: 1) life cycle economics and 2) materials system sustainability – particularly resource efficiency and recyclability. To date such methods, have been developed for and applied to issues associated with every major life-cycle stage.
Motivation
The 21st century presents a novel set of challenges to the engineer: intensive global competition, increasing carbon emissions, and a ballooning population drawing from a finite resource base. No engineer is better positioned to address these challenges than those who work with materials. Fundamentally, materials engineers manipulate matter to deliver new properties or develop novel processes to realize those forms of matter. However, materials are not simply a bundle of physical properties; materials influence the manner in which a product is fashioned, the form of that product, and, ultimately, its performance while in use. As a result, manipulating materials can change the nature of commerce, the interaction with the environment, and the character of resource-use. Materials can change the rules of the game.
The last several decades have seen the creation of robust analytical tools that map engineering decisions to their impact on technological performance. Moving forward, these tools will have to be complemented with ones that map to other characteristics. Dr. Kirchain’s research attempts to address this need for two such characteristics: (i) economic performance and (ii) environmental performance of materials technologies.
Mapping these properties is challenging because of the far-reaching implications of materials to the realization of a product; materials can change the economic and environmental characteristics of nearly every stage of the product life-cycle, including raw materials production, product design, manufacture, use, and disposal/recovery.
Dr. Kirchain’s research has shown that by building an integrated approach around the tools of engineering, economics, and operations research, it is possible to link materials decisions to their broader implications; it is possible to measure the economic and environmental performance of materials. To date, Dr. Kirchain has developed measurement methods that address each of the major product life-cycle stages (see figure below). His vision is that these methods will someday find their way into the toolkit of every graduating materials engineer and materials technology decision-maker.
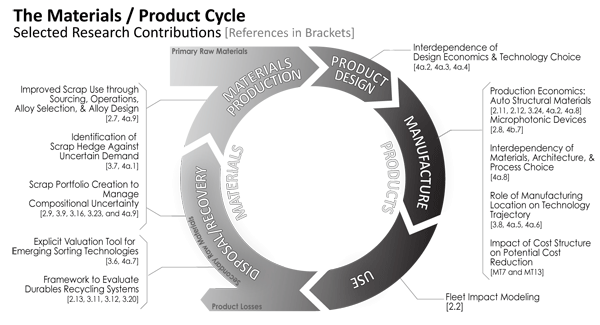
Research: Mapping the Broader Implications of Materials Design for Economic Performance
Economic Performance: Cost
On the face of it, cost appears to be a simple metric – the financial consequences of an action. However, when it comes to relating specific technical changes to their economic implications, cost can become a more elusive measure. The classical tools for analyzing costs are based in an accounting heritage which focuses on the performance of existing facilities and designs. This historical and aggregate focus limits the relevance of the resulting analyses when exploring significant technological change – the kind associated with novel materials technologies.
Fortunately, there are methods to meet this need. Specifically, Dr. Kirchain’s research group has been advancing the frontier of generative cost modeling methods. In the same way that engineering models allow designers to understand the physical consequences of their technical choices before implementation, generative cost models project economic consequences. A number of authors have applied generative cost models to study individual cases of technology selection or manufacturing configuration, but no common method has emerged. (Noble 1990; 1993) Dr. Kirchain’s research has focused both on applying generative models to explore case studies of materials technology competition and generalizing the specific method referred to as process-based cost modeling (PBCM).
Product Design
Within the context of structural materials selection, Dr. Kirchain’s group has demonstrated the application of the PBCM method to the process of product development. Such tools in concert with the manufacturing models mentioned above were used to (i) identify the potential for development costs to change with materials and, therefore, alter the materials selection decision, (ii) characterize the interdependence of materials choice and architectural platforming, and (iii) develop improved metrics to guide platforming decisions.
Manufacture
A core aspect of Dr. Kirchain’s case study work focuses on developing a comprehensive suite of process-based cost models to evaluate the competitive position of alternative structural materials within the automobile. This set of tools, in whole or in part, is used currently by General Motors, the DOE’s US Advanced Materials Partnership, and numerous materials suppliers and component producers throughout the automotive supply-chain. This work has identified the most economical opportunities for emerging materials such as advanced composites, high-strength steels, and light metals to reduce vehicle mass.
Additionally, Dr. Kirchain’s group applied PBCM to study the technologies of optoelectronic communications. Specifically, through a collaboration with Professors Lionel Kimerling and Rajeev Ram his group has developed the first detailed process-based cost model of optoelectronic component production. This model has shown that, contrary to widely held belief, it would be cost effective to utilize integrated designs even given current low production volumes. This analytical work served as a key element within the document Microphotonics: Hardware for the Information Age, the first-ever broad technology roadmap for the optoelectronic components industry that was published by the MIT Communications Technology Roadmap Project.
Through this work across two distinct industries, Dr. Kirchain’s research has developed generalizable modeling approaches to create models that are reconfigurable and scalable with regard to process flow and that can simultaneously analyze multiple platformed products. These tools now bring accurate information to the early stage of the decision process when materials technology choices are made.
In terms of fundamental learnings around the economics of materials technologies, these models have been applied to (i) characterize the impact of cost structure on expected cost evolution in response to manufacturing learning, specifically, identifying technologies with limited or great potential for cost decrease, (ii) demonstrate the tight coupling between materials, architecture, and process decisions, and (iii) identify the potential for manufacturing location to affect the technological trajectory of an industry.
Selected Publications:
General
F Field, R Kirchain, and R Roth, “Process Cost Modeling: Strategic Engineering and Economic Evaluation of Materials Technologies”, Journal of Metals, Vol. 49, Iss. 10, 2007. pp. 1543-1581.
doi: 10.1007/s11837-007-0126-0
R Kirchain and F Field, “Process-Based Cost Modeling: Understanding the Economics of Technical Decisions”, in Encyclopedia of Materials Science & Engineering, v2, pp 1718-27, Elsevier Science, 2000.
Product Design
M Johnson and R Kirchain, “Quantifying the Effects of Parts Consolidation and Development Costs on Material Selection Decisions: A Process-Based Costing Approach”, submitted to International Journal of Production Economics.
M Johnson and R Kirchain, “A Process-based Cost Modeling Approach to the Development of Product Families”, submitted to Journal of Product Innovation Management.
M Johnson and R Kirchain, “The Importance of Product Development Cycle Time and Cost in the Development of Product Families”, Journal of Engineering Design, Vol. 22, No. 2, 2011. pp. 87-99.
S Akamphon, “Methods for Capturing the Impact of Non-Steady State Production on Technology Choice”, Doctoral Thesis in Mechanical Engineering, June 2008.
M Johnson, “Quantifying the Impact of Material and Architecture on Development Cost”, Doctoral Thesis in Mechanical Engineering, June 2004.
Automotive
E Fuchs, F Field, R Roth, and R Kirchain, “Strategic Materials Selection in the Automotive Body: Economic Opportunities for Polymer Composite Design”, Composites Science and Technology, Vol.68, Iss. 9, 2008. pp.1989-2002.
doi: 10.1016/j.compscitech.2008.01.015
T Montalbo, T Lee, R Roth, and R Kirchain, “Modeling Costs and Fuel Economy Benefits of Lightweighting Vehicle Closure Panels”, SAE 2008 World Congress, Detroit MI, April 14-17, 2008, 12 pages.
S Matwick, “An Economic Evaluation of Sheet Hydroforming and Low Volume Stamping and the Effects of Manufacturing Systems Analysis”, Master’s Thesis, February 2003.
E Fuchs, “The Significance of Production Cost Inputs in Regional Technology Choice: Composite Automotive Body-In-Whites in the US versus China”, Master’s Thesis, Technology Policy Program, June 2003.
D Dantec, “Analysis of the Cost of Recycling Compliance for the Automobile Industry”, Master’s Thesis, Technology Policy Program, February 2005.
A Kar, “A Cost Modeling Approach Using Learning Curves to Study the Evolution of Technology”, Master’s Thesis, Materials Science and Engineering, June 2007.
R Cirincione, “A Study of Optimal Automotive Materials Choice Given Market and Regulatory Uncertainty “, Master’s Thesis, Technology Policy Program, June 2008.
Microphotonics
E Fuchs, E Bruce, R Ram, and R Kirchain, “Process-based Cost Modeling of Photonics Manufacture: The Cost Competitiveness of Monolithic Integration of a 1550-nm DFB Laser and an Electroabsorptive Modulator on an InP Platform”, Journal of Lightwave Technology, Vol. 24, Iss. 8, 2006, pp. 3175-3186.
doi: 10.1109/JLT.2006.875961
S Liu, “Process Based Cost Modeling of Emerging Optoelectronic Interconnects: Implications for Material Platform Choice”, Master’s Thesis, Technology Policy Program, June 2008.
Other industries
R Kirchain, The Role of Titanium in the Automobile: Understanding the Economic Implication of Three Emerging Technologies, prepared for United States Advanced Materials Partnership and the US Department of Energy. July 2002.
J Gregory and R Kirchain, “Eco-Efficient Recycling Alternatives for End-of-Life Cathode Ray Tubes”, Proceedings of the Annual Meeting of TMS: Light Metals Division, San Antonio, 2006, pp. 909-918.
J Gregory, J Atlee, and R Kirchain, “Driving Eco-Efficiency-Informed Decisions: A Process-Based Model of End-of-Life Electronics Recycling”, Proceedings of IEEE International Symposium on Electronics and the Environment, San Francisco, 2006, pp.138-143.
J Dahmus, S Fredholm, E Olivetti, J Gregory, and R Kirchain, “Modeling the Economic and Environmental Performance of Recycling Systems”, Proceedings of the IEEE International Symposium on Electronics and the Environment, San Francisco, CA, May 19-22, 2008, 6 pages.
Research: Mapping the Broader Implications of Materials Design for Environmental Performance
Environmental Performance
It is estimated that, in the US, total consumption of material resources has topped 60 kg per person per day. (Ayres 2003) While the average consumption of the rest of the world lags the United States by nearly an order of magnitude, it is growing at twice the rate. (Matos 1998) While these estimates are fraught with uncertainty, they clearly point to one of the significant challenges that confront engineers and scientists of the 21st century: how to deal with the deleterious effects associated with an unprecedented consumption of material resources. Concern over this issue is no longer limited to the isolated activist or green consumer. Firms, governments, and financial markets are all reorienting their approaches to industrial activity to account for environmental impact. Moving forward, materials technologies will also be judged by the burden they engender. (Bras 1997, Wegst and Asbhy 2002, Apelian 2007) To address this, a second focus of my research has been on developing tools to better characterize one specific aspect of environmental performance: recyclability.
Like cost, recyclability seems like a simple property, but in fact is dependent on the convolved characteristics of material, product, and recycling system. To deal with this challenge, Dr. Kirchain’s group and a few groups globally have developed mathematical programming and simulation models that can project the potential to recycle for arbitrary combinations of raw materials and desired alloy products. (van Schaik 2004; Reuter 2006; Rong 2008) Dr. Kirchain’s group has specifically demonstrated the potential for such tools to identify strategic opportunities to increase recyclability through changes in raw material sourcing, alloy-product matching, and alloy compositional modification.
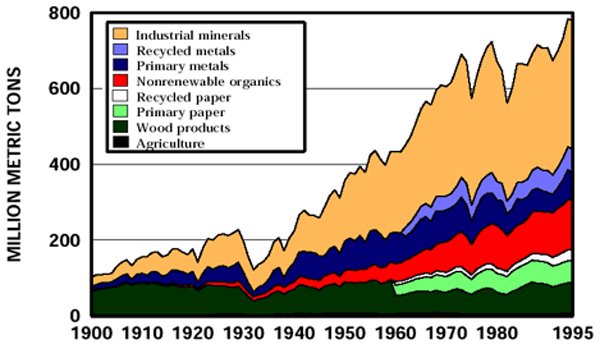
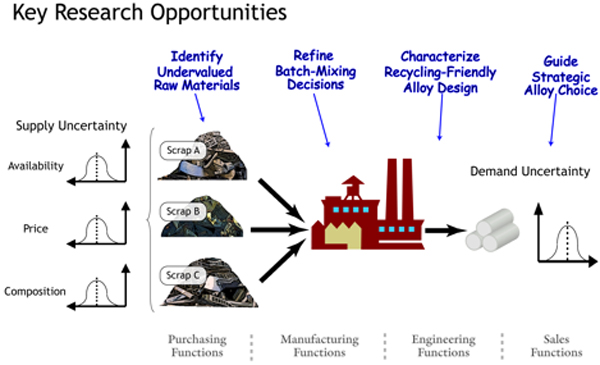
One technological strategy being explored to improve recyclability of many materials is sorting to improve scrap homogeneity. Although sorting is technically advantageous, its net economic value is unclear. Dr. Kirchain’s group has developed a coupled sorting and alloy production model to quantify that value and how it is affected by sorter efficiency and operational context. For a specific technology, the group has demonstrated that although improved sorter efficiency always improves cost and scrap use, it does not always translate into increased sorter utilization.
Considering Uncertainty
In an effort to make these models more reflective of real-world operations, Dr. Kirchain’s group has developed and implemented methods to incorporate the effects of uncertain demand and uncertain scrap composition into the decision process. In both cases, they learned that scrap use could be increased through the use of such stochastic models compared against conventional deterministic analyses. In the case of uncertain demand, this occurs because of the previously unrecognized hedging value of scrap. For composition, it emerges because traditional models do not manage variation by building up “scrap portfolios”. Currently, the focus is on generalizing these models so that they can be used for any materials recovery system (e.g., polymers, paper, glass) and even for the primary production of numerous biological materials (e.g., collagen, rubber).
Recycling Systems
Complementing the facility level modeling efforts around light metals, Dr. Kirchain’s group is carrying out a parallel research effort studying the performance of material recovery systems for complex durable goods (CDGs), most prominently end-of-life electronics. The initial focus of this work has been to characterize quantitatively the resource sustainability of such systems. Initial studies of six recycling facilities and the papers that followed developed novel, generalizable metrics of resource efficiency. Additionally, we have developed a generalizable model of a material recovery system for CDGs. This model can be used to explore the implications of policy and architectural decisions in terms of expected recovery and cost.
Selected Publications:
Recyclability
R Kirchain and A Cosquer, “Strategies for Maintaining Light Metal Reuse: Insights from Modeling of Firm-Wide Raw Materials Availability and Demand”, Resources, Conservation, and Recycling, Vol. 51, Iss. 2, 2007. pp. 367-396.
doi: 10.1016/j.resconrec.2006.10.005
G Gaustad, P Li, and R Kirchain, “Modeling Methods for Managing Raw Material Compositional Uncertainty in Alloy Production”, Resources, Conservation, and Recycling, Vol. 52, Iss. 2, 2007. pp. 180-207.
doi: 10.1016/j.resconrec.2007.03.005
P Li, S Guldberg, HO Riddervold and R Kirchain, “Identifying Economic and Scrap Reuse Benefits of Light Metals Sorting Technologies”, Proceedings of the Annual Meeting of TMS: EPD Congress 2005, San Francisco, 2005, pp 37-51.
The paper summarizing this work was selected as a Featured (Best) Paper at the TMS Annual Meeting 2005 in San Francisco, CA, one of seven out of 2500 papers.
P Li and R Kirchain, “Modeling Scrap Sourcing Decisions Given Uncertain Demand”, Proceedings of the Annual Meeting of TMS: Light Metals Division, San Francisco, 2005, pp 849-854.
G Gaustad, S Das, and R Kirchain, “Identifying Scrap Friendly Alloys Using Chance Constrained Modeling”, Proceedings of the Annual Meeting of TMS: Light Metals Division, Orlando, FL, February 25-March 1, 2007, pp. 1153-1160.
In 2006, this work was awarded the TMS Recycling Technology Award.
G Gaustad, R Peterson, and R Kirchain, “Modeling Methods to Guide Recycling Friendly Alloy Design: The Impact of Compositional Data Structure”, Proceedings of the Annual Meeting of TMS: 9th Global Innovations Symposium – Trends in Integrated Computational Materials Engineering for Materials Processing and Manufacturing, New Orleans, LA, March 9-13, 2008, pp. 263-270.
Recycling Systems
J Gregory and R Kirchain, “A Framework for Evaluating the Economic Performance of Recycling Systems: A Case Study of North American Electronics Recycling Systems”, accepted to Environmental Sciences and Technology, ASAP article released August 13, 2008, 9 pages.
doi: 10.1021/es702666v
J Gregory and R Kirchain, “Eco-Efficient Recycling Alternatives for End-of-Life Cathode Ray Tubes”, Proceedings of the Annual Meeting of TMS: Light Metals Division, San Antonio, 2006, pp. 909-918.
J Gregory, J Atlee, and R Kirchain, “Driving Eco-Efficiency-Informed Decisions: A Process-Based Model of End-of-Life Electronics Recycling”, Proceedings of IEEE International Symposium on Electronics and the Environment, San Francisco, 2006, pp.138-143.
J Dahmus, S Fredholm, E Olivetti, J Gregory, and R Kirchain, “Modeling the Economic and Environmental Performance of Recycling Systems”, Proceedings of the IEEE International Symposium on Electronics and the Environment, San Francisco, CA, May 19-22, 2008, 6 pages.
S Fredholm, J Gregory, and R Kirchain, “Characterizing Architectural Options for Electronic Waste Recycling Systems”, Proceedings of the IEEE International Symposium on Electronics and the Environment, San Francisco, CA, May 19-22, 2008, 6 pages.
E Olivetti, J Gregory, and R Kirchain, “Original Equipment Manufacturer End-of-Life Equipment Collection Metrics”, Proceedings of the IEEE International Symposium on Electronics and the Environment, San Francisco, CA, May 19-22, 2008, 6 pages.
Environmental Metrics
F Field, R Kirchain and J Clark; “Life Cycle Assessment and Temporal Distributions of Emissions: Developing a Fleet-Based Analysis”, Journal of Industrial Ecology, Vol. 4, Iss. 2, 2001, pp. 71-91.
doi: 10.1162/108819800569816
J Atlee and R Kirchain, “Operational Sustainability Metrics Assessing Metric Effectiveness in the Context of Electronics-Recycling Systems”, Environmental Science and Technology, Vol. 40, Iss. 14, 2006, pp. 4506-4513.
doi: 10.1021/es050935l
E Alonso, F Field, J Gregory, and R Kirchain, “Material Availability and the Supply Chain: Risks, Effects, and Responses”, Environmental Science and Technology, Vol.41, Iss. 19, 2007. pp. 6649-6656.
doi: 10.1021/es070159c
AN Allen, S Das, F Field, and R Kirchain, “Robustness of Materials Selection Decisions Using Various Life-Cycle Assessment Methods”, Proceedings of the TMS Conference on Materials Science and Technology (MS&T07), Detroit, Michigan, September 16-20, 2007, pp. 55-66.